June 8, 2025 2:32 pm
Functions of an Ecosystem
Ecosystems are incredibly complex, encompassing various processes that sustain life on Earth. Understanding these processes provides insight into how ecosystems maintain their stability and balance. The primary functions of an ecosystem can be divided into three major categories:
- Energy Flow: The transfer of energy through various trophic levels.
- Nutrient Cycling (Biogeochemical Cycles): The movement and recycling of nutrients between living and non-living components.
- Ecological Succession: The gradual development and change of ecosystems over time.
2.1 Energy Flow
- Definition: Energy flow refers to the transfer of energy from one trophic level to another within an ecosystem. This movement is unidirectional, meaning it flows in one direction: from the sun to producers and then to consumers.
- Importance: Energy is essential for all life processes, such as growth, reproduction, and maintenance of homeostasis. Without energy flow, ecosystems could not function.
2.2 Trophic Level Interaction
Ecosystems are structured based on the flow of energy, which is organized into different trophic levels. Each level represents a group of organisms that obtain their energy in a specific way.
- Trophic Levels:
- Level I: Autotrophs (Producers): Organisms like green plants, algae, and some bacteria that convert solar energy into chemical energy through photosynthesis. They are the foundation of the ecosystem, providing energy for all other organisms.
- Level II: Herbivores (Primary Consumers): These organisms consume producers to obtain energy. Examples include cows, deer, and insects that feed on plant matter.
- Level III: Carnivores (Secondary Consumers): Animals that feed on primary consumers. Examples include frogs, snakes, and small predatory fish.
- Level IV: Carnivores (Tertiary Consumers): Larger predators that feed on secondary consumers, such as wolves, eagles, and large fish.
- Level V: Top Carnivores (Quaternary Consumers): Apex predators that have no natural enemies. Examples include lions, sharks, and polar bears.
- Energy Transfer:
- Energy is transferred from one trophic level to the next, but with significant energy loss (around 90%) at each level, primarily in the form of heat. This loss limits the number of trophic levels in an ecosystem, usually to four or five.
- The 10% Rule: Only about 10% of the energy at one trophic level is passed on to the next level. For example, if a plant captures 1000 calories of solar energy, only about 100 calories are available to herbivores, and just 10 calories to carnivores.
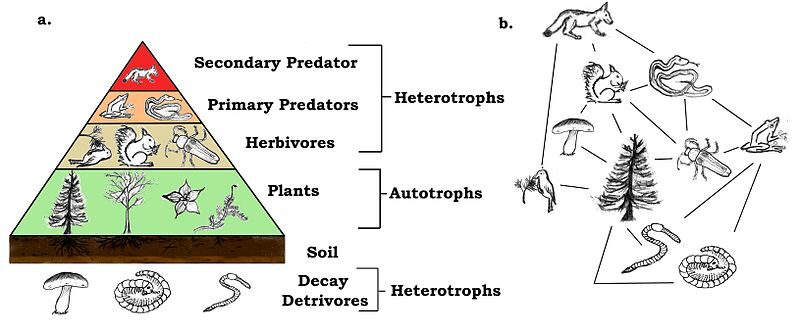
2.3 Concepts of Trophic Level Interaction
Food Chain:
A linear sequence of organisms where each organism is eaten by the next one in the chain. A sequence of organisms through which energy is transferred, starting from producers and ending with top predators.
- Characteristics: Simple and linear, with each organism serving as food for the next.
- Types of Food Chains:
- Grazing Food Chain:
- Explanation: Begins with producers (living plant biomass) and moves up to herbivores and then to carnivores.
- Example (Terrestrial): Grass → Grasshopper → Frog → Snake → Hawk
- Example (Aquatic): Phytoplankton → Zooplankton → Fish → Seal → Shark
- Detritus Food Chain:
- Explanation: Starts with dead organic matter, which is broken down by decomposers and then consumed by detritivores.
- Example: Dead leaves → Fungi → Earthworms → Birds
- Grazing Food Chain:
- Significance: Both types of food chains are interconnected and vital for nutrient recycling.
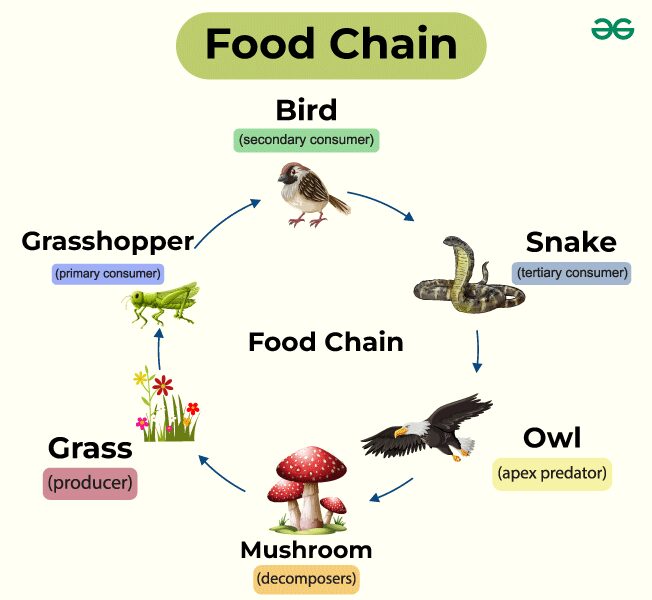
Food Web:
A more complex and realistic model of energy flow that consists of multiple interconnected food chains.
- Definition: A food web illustrates all possible feeding relationships and energy transfers within an ecosystem.
- Importance: It provides stability to the ecosystem by offering multiple pathways for energy flow, ensuring that organisms have alternative food sources if one species becomes scarce or extinct.
- Example: In a forest ecosystem, grass can be eaten by rabbits, deer, or insects. Each of these herbivores is preyed upon by different carnivores, such as foxes, hawks, and wolves. This interconnectedness forms a web rather than a single chain.
- Stability: If one species in a food web is removed, the ecosystem may be able to adapt and find balance through alternative pathways.
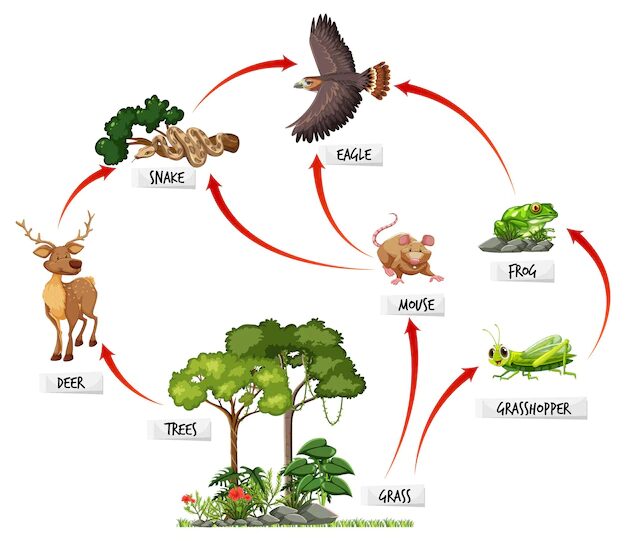
Ecological Pyramids:
This is a Diagrammatic representation of the structure and function of an ecosystem, illustrating the number, biomass, or energy of organisms at each trophic level.
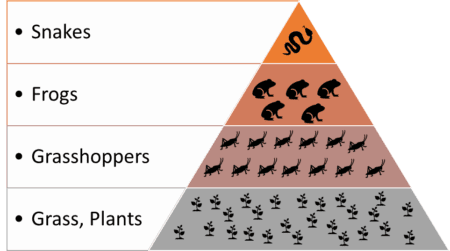
- Pyramid of Numbers: Shows the number of individual organisms at each trophic level. It can be:
- Upright: Seen in grassland and aquatic ecosystems, where many producers support fewer herbivores, which in turn support even fewer carnivores.
- Inverted: Seen in some forest ecosystems, where a few large trees (producers) support a larger number of herbivores and an even larger number of parasites or decomposers.
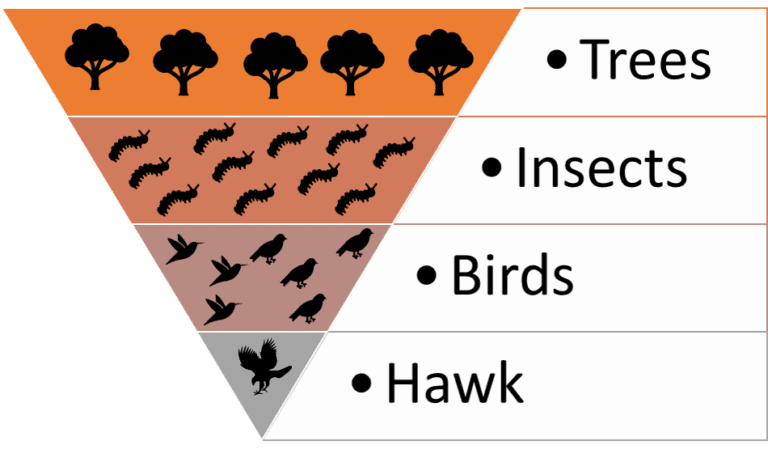
- Pyramid of Biomass: Illustrates the total mass of living material at each trophic level.
- Upright: Typical in terrestrial ecosystems, where biomass decreases as you move up the trophic levels.
- Inverted: Common in aquatic ecosystems, where the biomass of primary consumers (e.g., zooplankton) exceeds that of producers (e.g., phytoplankton) at any given time, because producers reproduce rapidly.
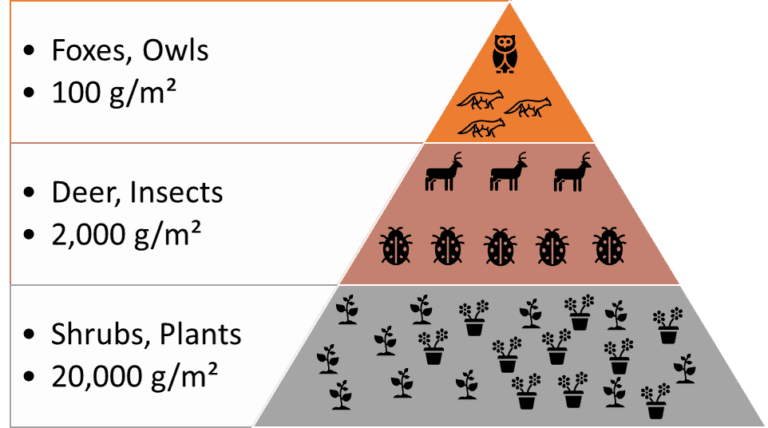
- Pyramid of Energy: Always upright, showing the energy loss at each trophic level. It reflects the inefficiency of energy transfer, as energy diminishes with each level.
- Example: If an ecosystem receives 10,000 calories of solar energy, only 1000 calories may be stored by plants, 100 calories by herbivores, and so on.
2.4 Pollutants and Trophic Levels
Pollutants are substances introduced into the environment that cause harm or adverse effects to ecosystems and living organisms. Some pollutants can be broken down naturally, but nondegradable pollutants are particularly concerning because they persist in the environment, accumulating over time and causing long-term damage.
Nondegradable Pollutants
- Definition: These are substances that cannot be broken down or decomposed by natural biological, chemical, or physical processes. Once introduced into an ecosystem, they remain in the environment for long periods, often becoming more concentrated as they move through trophic levels.
- Examples:
- Heavy Metals: Such as lead, mercury, and cadmium. These metals can contaminate water bodies, soil, and air, leading to toxic effects on wildlife and humans.
- Persistent Organic Pollutants (POPs): Chemical compounds like DDT or dichlorodiphenyltrichloroethane (a pesticide) and PCBs (polychlorinated biphenyls) that resist degradation and remain active in the environment.
Impact on Ecosystems
The presence of nondegradable pollutants has serious implications for ecosystems, especially when these substances become part of the food web. The impact is seen primarily through two processes:
- Bioaccumulation
- Definition: Bioaccumulation refers to the gradual accumulation of pollutants in the tissues of living organisms. This process occurs because these organisms absorb or ingest the pollutants faster than they can excrete or metabolize them.
- Mechanism: Pollutants like heavy metals and POPs (Persistent Organic Pollutants) enter organisms through water, food, or direct contact. As these substances are fat-soluble, they are often stored in fatty tissues, making them difficult to eliminate.
- Example: Fish in a contaminated water body may accumulate mercury in their tissues over time. Even though individual amounts of mercury may be small, the continuous exposure results in significant accumulation. Small aquatic organisms, like plankton, absorb mercury from the water. As these small organisms are eaten by slightly larger fish, the mercury accumulates in the tissues of the larger fish. This process continues up the food chain, with each predator consuming prey that contains higher concentrations of mercury
- Biomagnification
- Definition: Biomagnification is the process by which the concentration of pollutants increases as they move up the food chain. It occurs because each predator consumes numerous prey, each containing the accumulated pollutants, leading to higher concentrations in the predator.
- Mechanism: At each trophic level, the pollutant concentration becomes higher. Producers absorb pollutants from the environment. Herbivores that eat these producers accumulate higher levels of pollutants, and carnivores that eat the herbivores have even greater concentrations.
- Example:
- DDT: This insecticide was widely used to control pests but had severe ecological consequences. It accumulates in aquatic organisms like fish and magnifies in predatory birds like eagles and falcons. The high concentration of DDT in birds caused eggshell thinning, leading to decreased reproductive success and population decline.
- Mercury: Industrial waste often releases mercury into rivers and oceans, where it enters the food web. Small fish ingest mercury, and larger predatory fish like tuna accumulate higher levels. Humans who consume these fish are at risk of mercury poisoning, which can cause neurological and developmental problems.
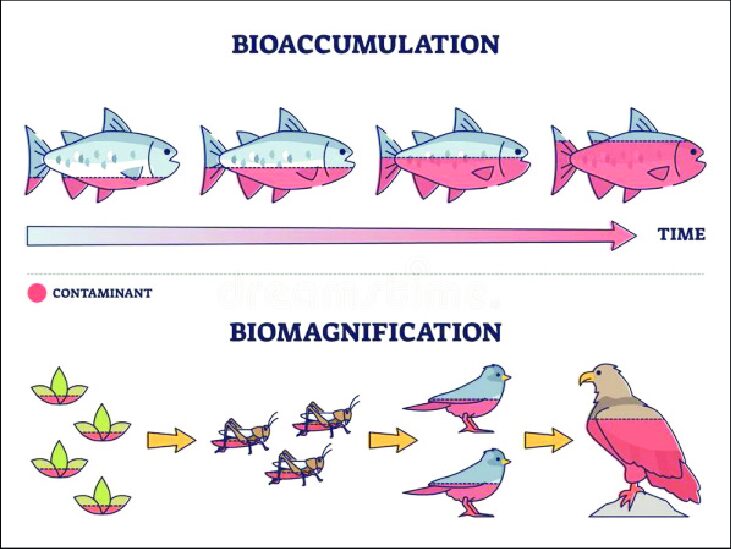
2.5 Biotic Interactions
The relationships and interactions between living organisms are fundamental to the functioning and stability of ecosystems. These interactions can be beneficial, harmful, or neutral, and they shape the distribution and abundance of species.
Definition
- Biotic interactions refer to the relationships among living organisms that influence their survival, reproduction, and population dynamics. These interactions are crucial for maintaining ecological balance and are categorized into several types based on the effects on the organisms involved.
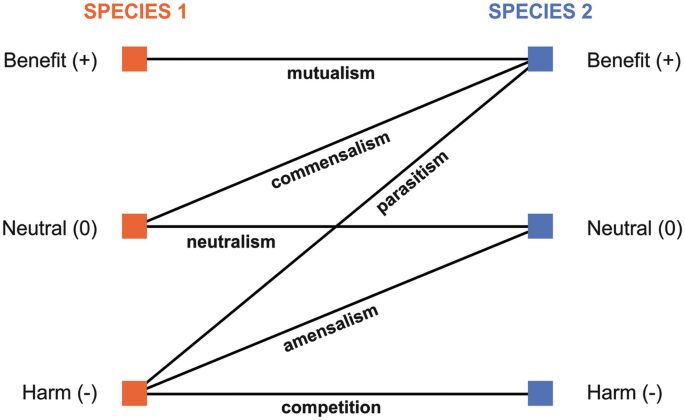
Types of Biotic Interactions
- Mutualism
- Definition: A type of interaction where both species benefit from the relationship.
- Examples:
- Bees and Flowering Plants: Bees collect nectar from flowers to make honey, while flowers get pollinated, allowing them to reproduce.
- Clownfish and Sea Anemones: The clownfish receives protection from predators by living among the sea anemone’s stinging tentacles, while the sea anemone benefits from the clownfish’s waste as nutrients.
- Commensalism
- Definition: An interaction where one species benefits, and the other is neither harmed nor helped.
- Examples:
- Barnacles and Whales: Barnacles attach themselves to the surface of whales. The barnacles get a free ride and access to nutrient-rich waters, while the whale remains unaffected.
- Epiphytes on Trees: Epiphytes, like orchids, grow on trees to receive better sunlight and air exposure. The trees are not harmed or benefited.
- Competition
- Definition: An interaction where both species are negatively affected because they compete for the same limited resources, such as food, water, or territory.
- Examples:
- Lions and Cheetahs: Both species compete for similar prey, such as antelope, in the African savannah. This competition can lead to reduced access to food and lower population numbers for both predators.
- Plants in a Dense Forest: Trees and plants compete for sunlight, water, and nutrients in a crowded environment. Some species may outcompete others, leading to reduced growth or death for the weaker competitors.
- Predation
- Definition: An interaction where one species (the predator) benefits by hunting, killing, and consuming another species (the prey).
- Examples:
- Wolves and Deer: Wolves hunt deer for food. The wolves benefit by gaining energy, while the deer population is kept in check, which can prevent overgrazing of vegetation.
- Owls and Mice: Owls hunt and eat mice. This interaction controls the mouse population and provides the owl with nourishment.
- Parasitism
- Definition: A relationship where one species (the parasite) benefits at the expense of the other (the host), often without killing the host.
- Examples:
- Ticks on Mammals: Ticks attach to mammals like deer or dogs and feed on their blood. The ticks gain nutrients, while the host may suffer from blood loss, irritation, or disease transmission.
- Tapeworms in Humans: Tapeworms live in the intestines of humans, absorbing nutrients from the host’s food. This can lead to malnutrition and other health issues for the infected person.
- Amensalism
- Definition: An interaction where one species is harmed while the other remains unaffected.
- Examples:
- Black Walnut Trees and Nearby Plants: Black walnut trees release a chemical called juglone from their roots, which inhibits the growth of nearby plants. The surrounding vegetation suffers, while the walnut tree remains unaffected.
- Antibiotic Production by Fungi: Some fungi produce antibiotics that kill or inhibit bacteria. The bacteria are harmed, but the fungi are unaffected by their presence.
- Neutralism
- Definition: A relationship where neither species is significantly affected by the interaction.
- Examples:
- Desert Animals: A lizard and a cactus in the same desert ecosystem might not interact or influence each other in any meaningful way.
- Coexisting Species: In a large ecosystem, many species may coexist without impacting each other, especially if they use different resources or live in separate niches.
2.7 Biogeochemical Cycles
- Definition: The cycles that recycle nutrients between the biotic (living) and abiotic (non-living) components of the ecosystem.
- Importance: These cycles ensure the continuous availability of essential elements for life.
Types of Biogeochemical Cycles
1. Water Cycle
The water cycle, or hydrologic cycle, describes the continuous movement of water within the Earth and atmosphere. It is powered by solar energy and involves several key processes:
- Processes:
- Evaporation: The transformation of water from liquid to vapor, driven by the sun’s heat. It mainly occurs in oceans, rivers, and lakes.
- Transpiration: The release of water vapor from plants into the atmosphere. Plants absorb water from the soil and release it through small pores in their leaves.
- Condensation: The process by which water vapor cools and changes back into liquid droplets, forming clouds.
- Precipitation: Water droplets in clouds combine to become heavy and fall to the ground as rain, snow, sleet, or hail.
- Runoff: Water flows over the surface of the ground and collects in rivers, lakes, and oceans, eventually evaporating back into the atmosphere.
- Importance:
- Water is essential for all living organisms, acting as a medium for biochemical reactions and helping transport nutrients and waste.
- It regulates the Earth’s temperature and climate, contributing to the formation of ecosystems and habitats.
- Storage: Water is stored in various reservoirs, including:
- Oceans: The largest reservoir, holding about 97% of Earth’s water.
- Glaciers and Ice Caps: Store most of the freshwater in frozen form.
- Groundwater: Water that seeps into the soil and is stored underground in aquifers.
- Atmosphere: Water vapor that contributes to weather and climate patterns.
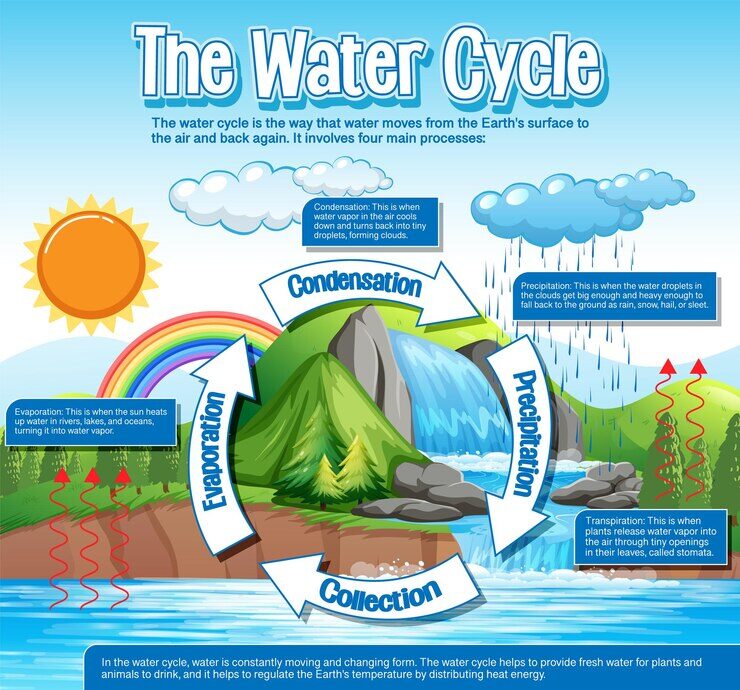
2. Carbon Cycle
The carbon cycle describes the movement of carbon between the atmosphere, living organisms, oceans, and the Earth’s crust. Carbon is a fundamental building block of life, forming the basis of organic molecules such as carbohydrates, proteins, and DNA.
- Processes:
- Photosynthesis: Plants, algae, and some bacteria absorb carbon dioxide (CO₂) from the atmosphere and use sunlight to convert it into glucose (a form of energy) while releasing oxygen.
- Respiration: Organisms release CO₂ back into the atmosphere as they break down glucose to produce energy.
- Decomposition: Decomposers, such as bacteria and fungi, break down dead organisms, releasing carbon back into the soil and atmosphere.
- Combustion: The burning of fossil fuels (like coal, oil, and natural gas) and organic matter releases stored carbon as CO₂ into the atmosphere.
- Importance:
- Carbon is crucial for forming organic molecules, making it vital for the structure and function of all living organisms.
- It plays a role in regulating the Earth’s climate and is stored in the atmosphere, oceans, and terrestrial ecosystems.
- Human Impact:
- The burning of fossil fuels and deforestation have increased atmospheric CO₂ levels, contributing to global warming and climate change.
- This has led to the greenhouse effect, where excess CO₂ traps heat in the atmosphere, causing temperature rises and impacting ecosystems.
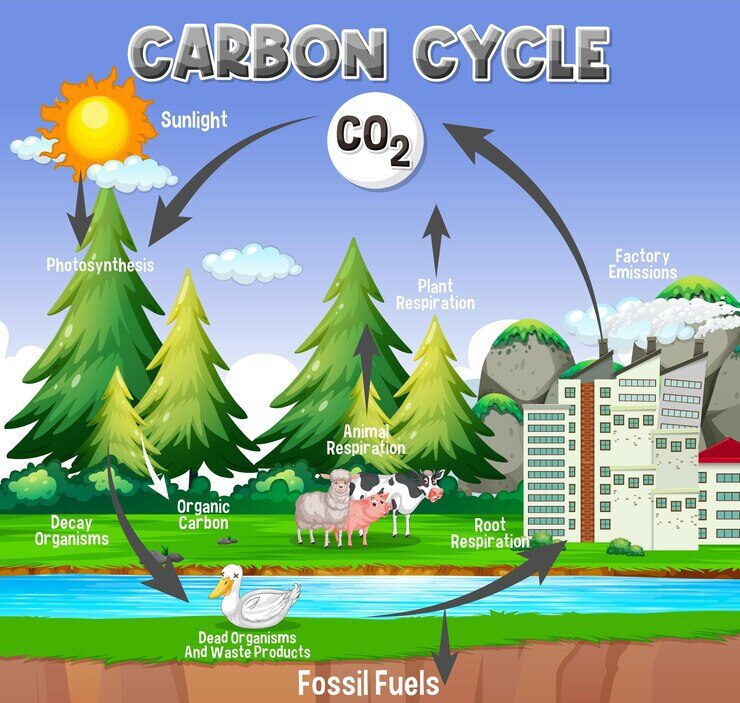
3. Nitrogen Cycle
The nitrogen cycle is essential for living organisms as nitrogen is a critical component of amino acids (proteins) and nucleic acids (DNA and RNA). However, nitrogen in its elemental form (N₂) is not usable by most organisms and must be converted into more accessible forms.
- Processes:
- Nitrogen Fixation: Specialized bacteria (e.g., Rhizobium) and blue-green algae convert atmospheric nitrogen (N₂) into ammonia (NH₃) or nitrates (NO₃⁻), which plants can use.
- Nitrification: Soil bacteria convert ammonia into nitrites (NO₂⁻) and then into nitrates (NO₃⁻), which are absorbed by plants.
- Assimilation: Plants take up nitrates from the soil and incorporate them into proteins and nucleic acids.
- Ammonification: Decomposers break down organic nitrogen from dead organisms and waste products, converting it back into ammonia.
- Denitrification: Certain bacteria convert nitrates back into nitrogen gas (N₂), releasing it into the atmosphere and completing the cycle.
- Importance:
- Nitrogen is vital for the growth and development of all organisms as it is a major component of proteins and DNA.
- It contributes to the productivity of ecosystems and agricultural systems.
- Nitrogen Fixation: Performed by nitrogen-fixing bacteria, this process is crucial for converting inert atmospheric nitrogen into a form that plants can use to grow.
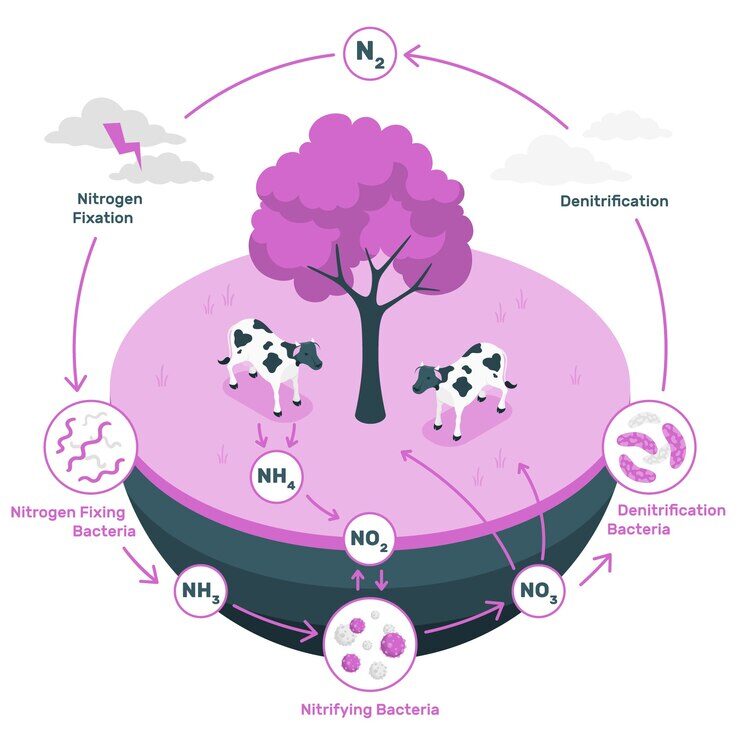
4. Phosphorus Cycle
The phosphorus cycle differs from other biogeochemical cycles because it does not have a significant gaseous phase. Phosphorus moves mainly through the lithosphere (Earth’s crust), hydrosphere (water bodies), and living organisms.
- Processes:
- Weathering of Rocks: Phosphorus is released from rocks and minerals as phosphate ions (PO₄³⁻) through weathering and erosion. These ions are carried into the soil and water bodies.
- Absorption by Plants: Plants absorb phosphate ions from the soil, incorporating them into organic molecules like DNA, RNA, and ATP.
- Consumption: Herbivores and carnivores obtain phosphorus by eating plants or other animals.
- Decomposition: When plants and animals die, decomposers return phosphorus to the soil or sediment, where it can be reused by plants.
- Sedimentation: In aquatic systems, phosphorus can settle in the form of sediments and may eventually form new rock layers, continuing the cycle.
- Importance:
- Phosphorus is a key nutrient needed for energy transfer (ATP), genetic material (DNA and RNA), and the structural integrity of bones and teeth.
- It plays a significant role in cell function and metabolism.
- Limitation: Phosphorus is often a limiting nutrient in ecosystems, meaning its availability can restrict the growth of organisms. It does not have a gaseous phase, so it cycles slowly, and its scarcity can impact ecosystem productivity.
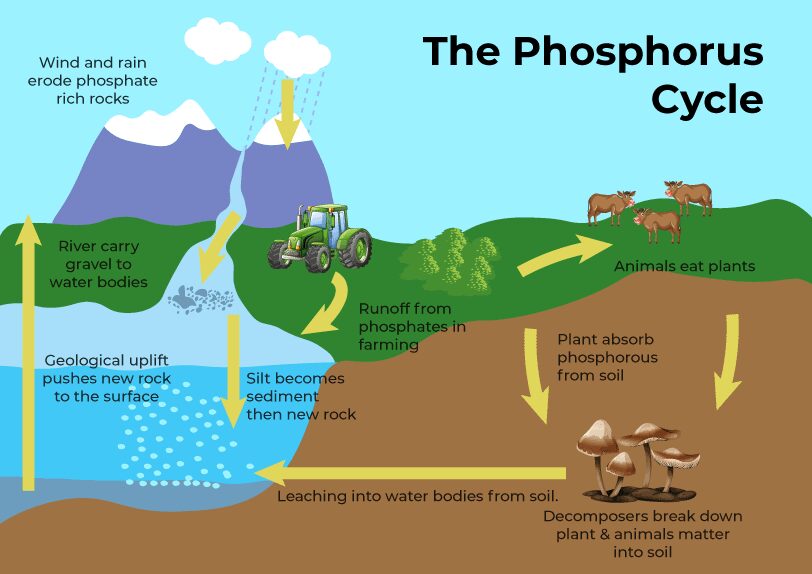
5. Sulfur Cycle
The sulfur cycle involves the movement of sulfur through the atmosphere, biosphere, and geosphere. Sulfur is a component of amino acids and vitamins and is essential for the health of organisms.
- Processes:
- Weathering: Sulfur is released from rocks and minerals through weathering and enters the soil and water bodies.
- Decomposition: When plants and animals die, sulfur is returned to the soil and is used by microorganisms.
- Volcanic Activity: Volcanoes release sulfur dioxide (SO₂) into the atmosphere, which can later return to the Earth’s surface through precipitation.
- Human Activities: The burning of fossil fuels and industrial processes release large amounts of sulfur dioxide into the atmosphere.
- Importance:
- Sulfur is a critical element in amino acids, proteins, and vitamins, contributing to various metabolic processes.
- It helps in the formation of proteins and the regulation of enzyme functions.
- Human Impact:
- Human activities, such as burning coal and oil, release sulfur dioxide into the atmosphere, leading to the formation of acid rain. Acid rain damages ecosystems, corrodes buildings, and acidifies water bodies, harming aquatic life.
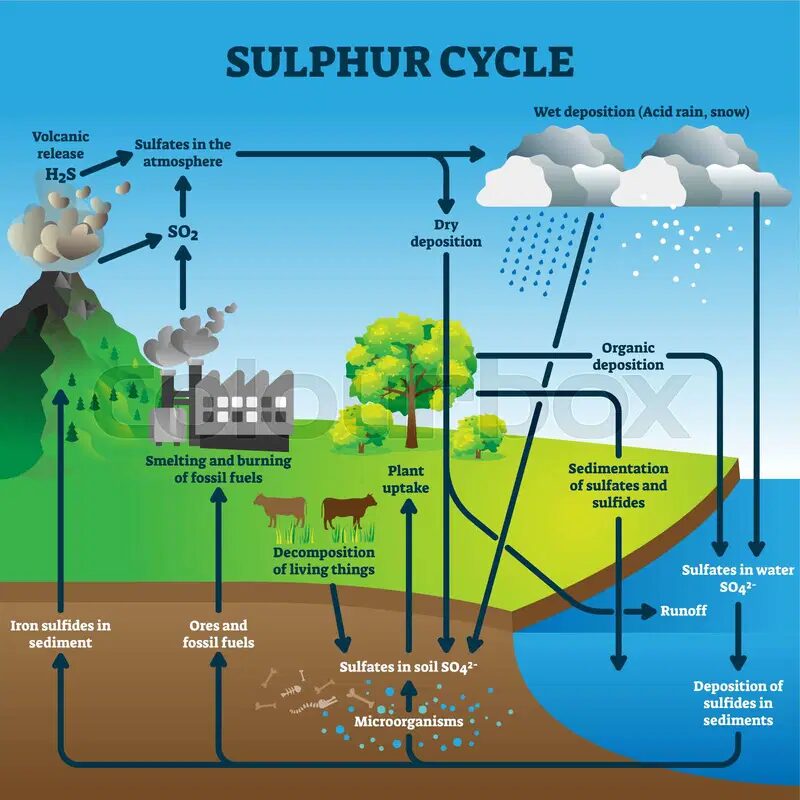
2.8 Ecological Succession
- Definition: The gradual change in an ecosystem’s species composition over time.
- Types: Primary and Secondary .Ecological succession refers to the gradual process through which ecosystems change and develop over time. It occurs in two main types: Primary Succession and Secondary Succession.
Primary Succession
- Definition: Primary succession occurs on newly formed or exposed land where no initial soil exists. This typically happens in places where a volcanic eruption creates new land or glaciers retreat, exposing bare rock.
- Pioneer Species:
- The first organisms to colonize the area are known as pioneer species. These are usually hardy species like lichens and mosses that can survive in harsh, barren conditions.
- Role of Pioneer Species: Lichens and mosses break down rocks through physical and chemical means, contributing to the formation of soil. As they decompose, they add organic material to the rock fragments, gradually creating a thin layer of soil.
- Process of Primary Succession:
- Colonization by Pioneer Species: Lichens and mosses establish themselves on the bare rock.
- Soil Formation: As pioneer species die and decompose, they create organic matter, which, combined with weathered rock, forms the first layer of soil.
- Arrival of Simple Plants: Grasses and small herbaceous plants begin to grow in the thin soil, further enriching it as they die and decay.
- Development of Shrubs and Small Trees: With more soil accumulation, shrubs and small trees establish themselves, providing habitats for animals and increasing the complexity of the ecosystem.
- Climax Community: Over hundreds or even thousands of years, a stable and mature climax community develops, which could be a dense forest or other types of vegetation, depending on the climate and conditions.
Example: A newly formed volcanic island or a landscape left behind by retreating glaciers.
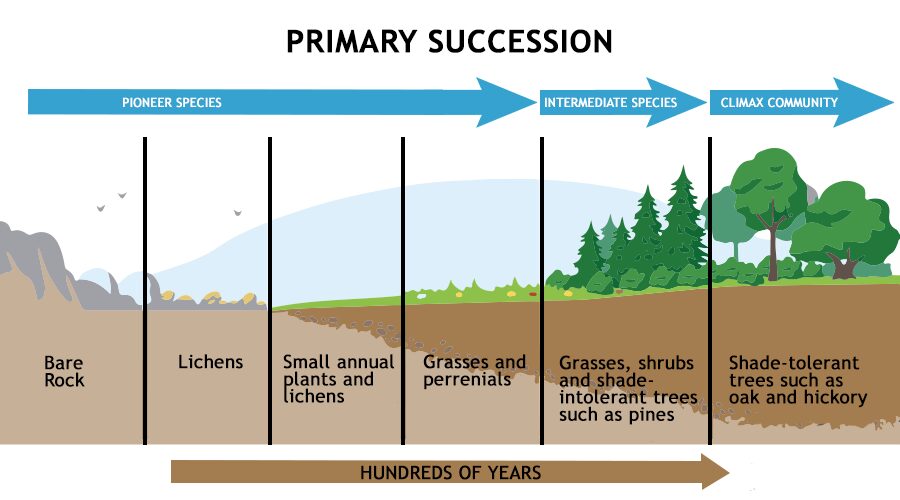
Secondary Succession
- Definition: Secondary succession occurs in areas where an existing community has been disturbed or destroyed but where soil and organic matter remain intact. This could be due to natural disturbances like forest fires, hurricanes, or human activities like deforestation or agricultural abandonment.
- Examples:
- Regrowth After a Forest Fire: In a forest that has been destroyed by fire, new vegetation begins to regrow from the remaining seeds, roots, and soil.
- Abandoned Agricultural Field: An abandoned farm field may gradually be colonized by grasses, shrubs, and eventually trees.
- Process of Secondary Succession:
- Initial Colonization: Fast-growing plants, such as grasses and wildflowers, quickly colonize the area due to the presence of existing soil and seed banks.
- Growth of Shrubs and Small Trees: As conditions improve, shrubs and small trees start to grow, attracting insects, birds, and small animals.
- Development of a Mature Forest: Over time, the ecosystem becomes more complex, and a mature forest or stable community emerges, depending on the environmental conditions.
- Speed: Secondary succession is generally faster than primary succession because the soil is already present, and seeds, roots, and organisms are often already in the environment. It can take decades to a few hundred years, compared to the thousands of years required for primary succession.
Example: A forest that regrows after being cleared for agriculture or a meadow forming on a previously logged area.
- Characteristics: Increased biodiversity, more complex food webs, and the formation of a stable climax community.
- Autogenic Succession: Driven by the living organisms in the community (e.g., soil formation by plants).
- Allogenic Succession: Driven by external factors like climate change or natural disasters.
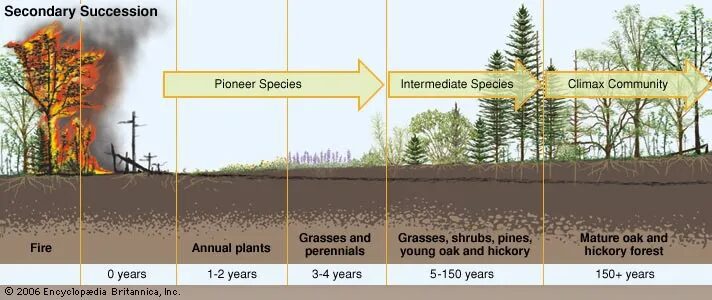
[…] Functions of an Ecosystem, Food Chain, Ecological Pyramids, Biogeochemical Cycles […]
[…] Functions of an Ecosystem Kyoto Protocol Climate Change and India […]
[…] Functions of an Ecosystem Terrestrial Ecosystems […]
[…] Functions of an Ecosystem Terrestrial Ecosystems Ecotone and Biome […]
[…] Functions of an Ecosystem Terrestrial Ecosystems Wetland ecosystem and Conservation Ecotone and Biome Convention on Biological Diversity […]